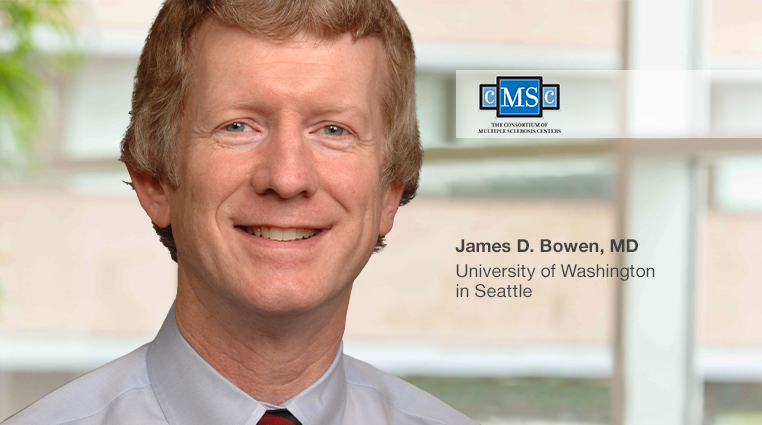
Multiple sclerosis (MS) is a complicated demyelinating disease of the central nervous system, where patients experience a great range of symptoms due to demyelination of nerve fibers. It is characterized by plaques in the brain and spinal fluid. Symptoms that have been observed include numbness, diplopia, cognitive impairment, paralysis, blindness, fatigue, and bladder dystonia. [1]
It is the most common disabling neurological condition affecting young adults around the world, and most commonly diagnosed between the ages of 20 and 40 years of age. In the US, there are over 400,000 people affected with MS and approximately 200 new cases are being diagnosed every week. The incidence is higher further from the equator and on colder climates, People with Northern European ancestry tend to have the highest incidence no matter where they live. Women tend to be more affected than men at a 2:1 ratio. [2] While MS has long been considered an autoimmune disease, the exact cause remains unknown.
In MS, it appears that the myelin sheath and nerve fibers are destroyed by immunological factors. Elevated levels of Immunoglobulin-G (Ig-G) have been detected in the CSF of MS patients. Deposits of immunoglobulin and complement have been detected in a subset of MS patients but it is unclear how they access the parenchyma. In studies with mice, it was shown that antibodies were deposited via paravascular route in the subarachnoid and subpial space as well as well as in the periventricular region. Researchers further found a complement-dependent loss of the integrity of the BBB at certain sites in the brain parenchyma. It is further theorized that AQP4/NMO-IgG enters through BBB-deficient sites where CSF access occurs. [3]
Cognitive deficits have been seen in approximately 43 to 65 percent of MS patients, most commonly memory alteration and thought processing speed. To a lesser degree, patients with MS have demonstrated deficits in visuospatial abilities and executive functioning. These findings have been strongly correlated with white matter lesion volume and brain atrophy. [4]
In the early stages of MS, cerebral subcortical deep gray matter nuclei (DGM) is a common area of atrophy. Atrophy in these areas is also a common feature of progression variations of MS. In one study utilizing MRI imagining, clinically significant atrophy was found in DGM in MS patients. Additionally, it was found that 1.5T and 3T were not interchangeable in measuring brain volumes, with 3T showing slightly better differentiation. The exact cause of DGM in MS is unknown but possible suggestions include iron deposits, oxidative stress, direct injury by demyelinating lesions, and Wallerian degeneration. [5]
It is generally agreed that MS is an inflammatory process that is triggered by environmental factors in patients who are genetically susceptible. Numerous susceptible genome wide association studies (GWAS) have identified susceptible loci, with the human leukocyte antigen (HLA) immune gene cluster, especially HLA-DRB1*1501, being the main genetic risk factor. In view of isolated outbreaks and migration studies, it has long been postulated that there is an infectious component to MS, with viruses being the major suspect. Many researchers believe that an endogenous retrovirus may play a major role in the development of MS. [6]
One interesting observation in neuropathological studies from the brain samples of MS patients is the fact that features of acute inflammation are rarely found in patients with progressive disease. Rather, they are found to have smoldering, chronic lesions with microglial activation near the edge of a burned-out plaque. Microglial activation has been associated with neuronal damage and atrophy. [7]
Many recent studies have shown that microglia have both neuroprotective and neurotoxic effects. In fact, microglia have been shown to produce certain inflammatory cytokines, including Interleukin 1 beta (1L-1B), tumor necrosis factor 1 (TNF1), Interleukin 6 (IL6), superoxide, nitric oxide, and excitatory amino acids. Neuroprotective factors that are produced include neurotrophins, Brain-derived Neurotrophic factor (BDNF), glial cell-derived neurotrophic factor (GDNF), and nerve growth factor (NGF). When activated, microglia produce CNS-infiltrating monocyte-derived macrophages, which upregulate many surface molecules. In cases of neuroinflammation and neurodegeneration, aggregation of microglia is seen. Once there are considerable CNS lesions seen, damage-associated molecular patterns (DAMPs) are released, leading to significant alterations in gene expression. [8]
While gray matter alterations are well known in MS, white matter dysfunction remains a hallmark of this disease. The damage is most notably seen in the corticospinal, superior longitudinal fasciculus, periventricular, and corpus callosum tracts. Task related MRI has shown that patients with MS show dysfunction in brain structure, brain activity and connectivity and these changes directly alter motor function. [9]
Great advances have been made in understanding brain pathology in MS patients in recent years. However, we need more understanding in order to create medications that targets those disturbances.
Want to learn more about our programs on Multiple sclerosis, in neurology, and beyond? Email us!
About the Author
Linda Girgis MD, FAAFP is a family physician practicing in South River, New Jersey and Clinical Assistant Professor at Rutgers Robert Wood Johnson Medical School. She was voted one of the top 5 healthcare bloggers in 2016. Follow her on twitter @DrLindaMD.
1. Phyo AZZ, Demaneuf T, De Livera AM, et al. The Efficacy of Psychological Interventions for Managing Fatigue in People With Multiple Sclerosis: A Systematic Review and Meta-Analysis. Frontiers in Neurology. 2018;9:149. doi:10.3389/fneur.2018.00149.
2. Multiple Sclerosis: Statistics, Facts and You. Healthline. https://www.healthline.com/health/multiple-sclerosis/facts-statistics-infographic#4. Accessed May 3, 2018.
3. Mørch MT, Sørensen SF, Khorooshi R, Asgari N, Owens T. Selective localization of IgG from cerebrospinal fluid to brain parenchyma. Journal of Neuroinflammation. 2018;15:110. doi:10.1186/s12974-018-1159-8.
4. Masuda H, Hirano S, Takahashi N, et al. Comparison of cognitive and brain grey matter volume profiles between multiple sclerosis and neuromyelitis optica spectrum disorder. Suzumura A, ed. PLoS ONE. 2017;12(8):e0184012. doi:10.1371/journal.pone.0184012.
5. Chu R, Hurwitz S, Tauhid S, Bakshi R. Automated segmentation of cerebral deep gray matter from MRI scans: effect of field strength on sensitivity and reliability. BMC Neurology. 2017;17:172. doi:10.1186/s12883-017-0949-4.
6. Bhetariya P, Kriesel J, Fischer K. Analysis of Human Endogenous Retrovirus Expression in Multiple Sclerosis Plaques. Journal of emerging diseases and virology. 2017;3(2):10.16966/2473-1846.133.
7. Airas L, Nylund M, Rissanen E. Evaluation of Microglial Activation in Multiple Sclerosis Patients Using Positron Emission Tomography. Frontiers in Neurology. 2018;9:181. doi:10.3389/fneur.2018.00181.
8. Mammana S, Fagone P, Cavalli E, et al. The Role of Macrophages in Neuroinflammatory and Neurodegenerative Pathways of Alzheimer’s Disease, Amyotrophic Lateral Sclerosis, and Multiple Sclerosis: Pathogenetic Cellular Effectors and Potential Therapeutic Targets. International Journal of Molecular Sciences. 2018;19(3):831. doi:10.3390/ijms19030831.
9. Peterson DS, Fling BW. How changes in brain activity and connectivity are associated with motor performance in people with MS. NeuroImage : Clinical. 2018;17:153-162. doi:10.1016/j.nicl.2017.09.019.